3. Design of the Tube Belt
Takraf ordered an approximately 9.1 km long tube belt from ContiTech Conveyor Belt Group for the new Maritza tube conveyor system in Bulgaria. ContiTech also helped during the planning, installation and commissioning of the system. They have supplied more than 120 tube belts worldwide since 1987 and enjoy a high level of trust and confidence both from OEMs and from many plant operators.
In comparison to a conventional troughed overland conveyor belt, which can be operated at maximum conveyor speeds of vF = 8.0…8.5 m/s, the maximum conveyor speed of a tube conveyor system is limited to around vF = 6.5 m/s. Furthermore, the conveyor cross-section of a troughed conveyor belt of equal width is around 3 times larger. However, a tube conveyor belt offers decisive advantages over a troughed conveyor belt, and in some cases these advantages make the use of a tube conveyor belt actually indispensable. A summary of the advantages and disadvantages of a tube conveyor system is outlined in Table 1.
Advantages: Tube vs. Trough Conveyor | Disadvantages: Tube vs. Trough Conveyor |
---|---|
Tight horizontal & vertical 3D-curve radii easily conforms to terrain | Higher energy consumption |
Steep conveyance angles up to 35° possible | Higher operation and maintenance costs |
No need for transfer points | Higher manufacturing and installation costs |
Material conveyed is shielded from outside environment | Lower capacity |
Minimal space required thanks to compact design | Special knowledge is required for design, installation, commissioning & operation |
Minimal spillage of material along conveyor structure |
During the selection and design of a tube conveyor and the tube belt itself, many factors need to be taken into account, such as the radii of curved sections, the significantly increased running resistance caused by the high form forces and additional friction forces in the belt overlaps, the shape of the overlap zone, and so on. This is why a new tube conveyor and the tube belt are often jointly designed by a OEM and a conveyor belt manufacturer. The new Maritza tube conveyor uses a high speed tube belt “HS-Rollgurt 1500 S-K2 7T:6S Conti Extra” with a width of 1500 mm and a nominal breaking strength of kN = 1500 N/mm. Figs. 6 to 8 present the special features and requirements associated with a tube belt.
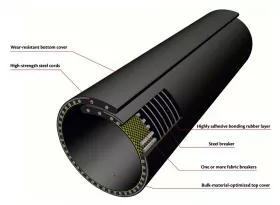
The highest friction forces in the conveyor system occur during the initial hours (days) when the tube belt is first taken into operation (i.e. empty) (exception: conditions when the belt is first pulled in). The design of a tube belt should always be based on a “normal operating state” (i.e. after the running-in period), while the choice of suitable drives should always take into account both possible operating states (i.e. during commissioning and after the running-in period).
If a tube belt is designed, selection of the optimal transverse stiffness and overlap design is essential. Fig. 7 shows a different behaviour of a tube belt between idler panels: good (left) and unsuccessful designs of the tube belt (middle and right). Fig. 8 shows a different behaviour of a tube belt under tension in a horizontal curve: sufficient (left) and non-sufficient (right) transverse stiffness.


Once the tube belt design has been decided upon, the forming forces and overlapping zone are tested with the aid of a test sample (Fig. 9). Various operating factors such as the ambient temperature, temperature of the material, and the minimum radius for curved sections need to be taken into account here. Optimized transverse stiffness should maintain the tubular shape in curves to provide sufficient tube cross sectional area for the material and not cause very high running resistances resulting in high power demand.

4. Pulling in the Belt
The process for pulling in a tube belt needs to be coordinated between the OEM, belt manufacturer, service company and end-user. The pulling in procedure should be perfectly matched to the system.
The following factors need to be taken into account:
- The forces required to pull in the belt should be calculated in advance and monitored during the pulling-in process.
- Appropriate tools, machinery and auxiliary equipment matched to the demands of the system must be made available on-time (dozers, cable winches, crane, fork-lift truck, special pulling-in devices, connecting tools, splice shed, powered belt reeler, etc.).
- The optimum timing for pulling in the belt needs to be coordinated with the customer (special measures may be required if it is particularly cold).
Before start to pull in, a preliminary installation meeting between all participants should be held on site. The following strategies are possible for pulling in the belt:
- The entire tube belt can be pre-spliced next to the system (e.g. at the tail end or at the head end) and then drawn into the system.
- Each section in turn can be drawn in or counter the conveying direction from one particular position or from multiple positions in the system in the top strand and/or bottom strand.
- During the pulling-in of the belt, it is possible to use drive(s), dozer(s), cable winch(es) or a take-up device (either alone or in combination).

Fig. 10 shows the final strategy chosen for pulling in the belt.
The standard procedure for replacement of a tube belt involves the use of drives in order to reduce the very high pulling forces at the cable winch (dozer) or on the powered belt reeler. In this case the belt is pre-spliced and laid down next to the installation in order to reduce downtime. Drives are often not possible to use in a new installation. Here, the belt is pulled in sections that are connected one after the other. This also reduces the very high pulling force. The choice of the correct location in the system from which the belt can be pulled in is of paramount importance. Ideally, the system should have no curved sections and no elevations at this point. Figs. 11 and 12 show the special equipment used to pull in the belt.

The cone-shaped belt puller (clamp) allows the belt to be pulled in smoothly through the hexagonal idler stations in the system. The rolled-up tube belt is fixed form-locked in the pulling-in clamp between two steel cones. Here, the eye bolt of the inner cone must be able to rotate freely around its axis so that rotations on a pull rope that is not free of twisting can be compensated for.

In order to reduce the form forces of the tube conveyor at the start, packaging strapping (yellow) was wrapped around the first 5 m of the belt (0.5 m each). If necessary, further binding straps can be attached to the tube belt. In the area of the pulleys and/or the flat-to-troughed and troughed-to-flat transition zones, a conventional, flat puller (clamp) is used. Here, the pulley of the system must be protected against a tensioned pull rope with special pulley protection attachments. The pulling-in table with adjustable idlers seats and finger idlers has a very special design.
The process of pulling in the belt was performed in “creep mode” with a speed of vP = 4…5 m/min using a pulling dozer, a stationary dozer and a cable idler pulley system. A special beam with cable idler pulleys was used to pull in the belt at high elevations of the system. At the head of the system, the belt was pulled in with the aid of the already installed take-up. At the same time, a sliding additive was continuously fed in at the pulling-in table in the overlapping zone of the tube belt in order to reduce friction forces between the rubber belt edges of the overlap.
Fig. 13 shows the pulling in process at ground level and at an elevated point in the system.

During the process of pulling in the belt, the camber angle of the idlers was adjusted to ensure that the belt overlaps remained in the desired twelve o’clock position for the top strand and in the six o’clock position for the bottom strand. As a rule, there were around 16 idler seats on which the idlers need to be realigned. If the position of the overlap changed, it was necessary to turn back the tube belt. The low ambient temperatures resulted in high belt tensions and also caused additional difficulties due to snow and ice on the idlers and belt.
Special attention was paid to the technology used for splicing of the different sections. By using a special splice design, it is ensured that the same tube belt characteristics (such as optimal transverse stiffness, belt overlap, etc.) found in undisturbed sections of the belt are also provided at splices. All splicing work was supervised by an experienced ContiTech-supervisor on site.
5. Commissioning

Commissioning of the Maritza tube conveyor took place in the spring of 2010. Similar to the process of pulling in the belt, the tube belt was carefully monitored at the critical points during commissioning (particularly in curved sections and the flat-to-troughed and troughed-to-flat transition zones). The commissioning process for a tube belt system is normally accompanied by very high running resistances, which are caused by high form forces during the run-in period of the tube belt and by high friction forces in the overlapping area. As a result, an increased power demand needs to be taken into account when designing the drive power of the conveyor system.
In order to reduce the high friction forces in the belt overlap, a sliding additive (e.g. talcum powder) was added in the belt overlapping zone. Corrective measures were immediately put in place if the tube belt displayed any twisting (Fig. 14).
6. Power Consumption

Following an approximately 6-month test operation – a time span resulting from the start-up cycle of the power plant – measurements were taken to determine the power consumption of the TC-3A.
At peak throughputs of 1000 t/h, the power plant was able to provide a maximum average loading of the entire conveyor length of approx. 700 t/h. Fig. 15 (left) shows the individual measurements as measuring points to which a trend line was added.
Of particular note here is that there is no significant difference between idle power and the driving power of the loaded belt. If the fictitious friction coefficient ƒ is calculated back from the individual measurements, the following image results in Fig. 15 (right).
Idling gives rise to a fictitious friction coefficient that significantly exceeds the value for the loaded belt. This is caused by the aforementioned additional components of main resistance. These are largely independent of the loading state and determined primarily by the belt properties.
The design of the tube conveyor must take this effect into account. Particularly for small throughputs and/or conveyor goods with small bulk densities, the idle behavior of the conveyor belt largely determines the power requirement.
The conveyor belt was designed, as described above, with a fictitious friction coefficient of ƒ = 0.043. When the trend line shown above is extended to the finally estimated throughput of 1400 t/h, a fictitious friction coefficient of around 0.044 results. This is affirmation of the installed driving power of 2000 kW.
■